CFTR is a member of the C subfamily of the ABC transporter (ABC-T) superfamily of proteins [1, 2], [3]. Almost without exception, ABC-Ts are transmembrane proteins that use the energy of ATP binding and hydrolysis to accomplish the active import or export of various substrates across membranes [4]. Indeed, CFTR bears ATPase activity [5, 6], but biophysical methods have firmly demonstrated that CFTR, unique among ABC proteins, functions as a phosphorylation-activated and ATP-gated ion channel whereas its closest ABCC relatives function as multi-specific exporters of organic anions [7-10]. The long-range goal of this project is to understand how CFTR function and pharmacology are impacted by membrane lipids, with current focus on sphingomyelin (SM) and cholesterol [11, 12]. Because CFTR bears ion channel activity we can study it at excruciating functional detail, compared to typical transporters, which provides opportunities to understand how membrane lipids impact many other ABC-Ts.
Our published work has shown that function of wildtype CFTR is regulated by SM-mediated signaling [13-15] and by cholesterol [16]. SM and cholesterol are two important components of lipid rafts found in apical plasma membranes (PMs) of airway epithelial cells. CFTR protein shifts into lipid raft “clusters” rich in cholesterol and SM in response to activation, and shifts into platforms that are high in ceramides (CDs), the prominent sphingomyelin metabolite, under pathological conditions such as airway infection by bacterial or viral pathogens. However, it is not clear how this affects CFTR function or pharmacology [17, 18].
The McCarty lab has studied both components of this regulation of CFTR function by cholesterol and SM. We showed that exposure of CFTR-expressing airway epithelial cells or Xenopus oocytes to bacterial sphingomyelinase (SMase) leads to complete loss of channel function without removal of CFTR protein from the plasma membrane. This suggests that CFTR is shifted into a “silent” conformation by either loss of SM or gain of CD or its metabolites. We also showed that depletion of cholesterol from the plasma membrane in both airway epithelial cells and Xenopus oocytes led to alterations in CFTR channel function, including gating, permeation, and sensitivity to pharmacological potentiation, without complete loss of activity. Thus, it appears that the interactions of cholesterol and SM/CD play separate, non-complementary roles in regulating CFTR channel function, since cholesterol depletion only alters function and potentiator pharmacology while exposure to SMase leads to channels that can no longer be activated or potentiated. However, the precise mechanisms by which cholesterol and SM/CD regulate CFTR function have yet to be determined. While lipids are found in recent Cryo-EM structures of CFTR (Fig. 1), it is not known how or where on the CFTR protein either cholesterol or SM/CD and their metabolites bind to have these functional effects. Furthermore, because cholesterol appears to similarly regulate function of multiple ABC-Ts, including a close relative of CFTR that does not bear channel function [19, 20], this project may lead to insights that are generalizable across the broad ABC-T superfamily.
This project seeks to understand how membrane lipids affect CFTR function and pharmacology by applying a combination of electrophysiological, bioinformatic, and computational approaches (Fig. 2). It takes advantage of unique collaborations with Gumbart at Georgia Tech, a computational biology expert who has published with McCarty, and Strickland at Mercer University, a biochemistry instructor who trained with McCarty. This assembly of expertise helps ensure accomplishing the project goals.
Goal 1: Identify the mechanisms underlying regulation of CFTR function and pharmacology by PM cholesterol and phospholipids, and associated binding sites.
Goal 2: Identify the role of the lipid environment on CFTR structure and dynamics.
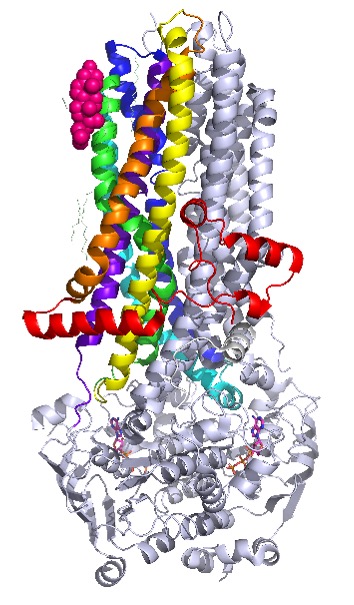
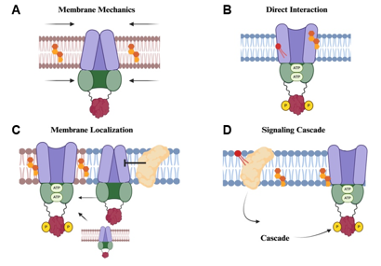
References
- Jordan, I.K., K.C. Kota, G. Cui, C.H. Thompson, and N.A. McCarty (2008) Evolutionary and functional divergence between the cystic fibrosis transmembrane conductance regulator and related atp-binding cassette transporters. Proceedings of the National Academy of Sciences of the United States of America. 105: 18865-70.
- Infield, D.T., K.M. Strickland, A. Gaggar, and N.A. McCarty (2021) The molecular evolution of function in the cftr chloride channel. The Journal of general physiology. 153: e202012625.
- Stratford, F.L., M. Ramjeesingh, J.C. Cheung, L.J. Huan, and C.E. Bear (2007) The walker b motif of the second nucleotide-binding domain (nbd2) of cftr plays a key role in atpase activity by the nbd1-nbd2 heterodimer. The Biochemical journal. 401: 581-6.
- Rees, D.C., E. Johnson, and O. Lewinson (2009) Abc transporters: The power to change. Nature reviews. Molecular cell biology. 10: 218-27.
- Li, C., M. Ramjeesingh, W. Wang, E. Garami, M. Hewryk, D. Lee, J.M. Rommens, K. Galley, and C.E. Bear (1996) Atpase activity of the cystic fibrosis transmembrane conductance regulator. The Journal of biological chemistry. 271: 28463-8.
- Cui, G., K.M. Strickland, A.J. Vazquez Cegla, and N.A. McCarty (2024) Comparing atpase activity of atp-binding cassette subfamily c member 4, lamprey cftr, and human cftr using an antimony-phosphomolybdate assay. Front Pharmacol. 15: 1363456.
- Anderson, M.P., H.A. Berger, D.P. Rich, R.J. Gregory, A.E. Smith, and M.J. Welsh (1991) Nucleoside triphosphates are required to open the cftr chloride channel. Cell. 67: 775-84.
- Anderson, M.P., R.J. Gregory, S. Thompson, D.W. Souza, S. Paul, R.C. Mulligan, A.E. Smith, and M.J. Welsh (1991) Demonstration that cftr is a chloride channel by alteration of its anion selectivity. Science. 253: 202-5.
- Bear, C.E., C. Li, N. Kartner, R.J. Bridges, T.J. Jensen, M. Ramjeesingh, and J.R. Riordan (1992) Purification and functional reconstitution of the cystic fibrosis transmembrane conductance regulator (cftr). Cell. 68: 809-818.
- Berger, H.A., M.P. Anderson, R.J. Gregory, S. Thompson, P.W. Howard, R.A. Maurer, R. Mulligan, A.E. Smith, and M.J. Welsh (1991) Identification and regulation of the cystic fibrosis transmembrane conductance regulator-generated chloride channel. The Journal of clinical investigation. 88: 1422-31.
- Cottrill, K.A., C.M. Farinha, and N.A. McCarty (2020) The bidirectional relationship between cftr and lipids. Commun Biol 3: 179.
- Farinha, C.M., E. Miller, and N. McCarty (2018) Protein and lipid interactions - modulating cftr trafficking and rescue. Journal of cystic fibrosis : official journal of the European Cystic Fibrosis Society. 17: S9-S13.
- Stauffer, B.B., G. Cui, K.A. Cottrill, D.T. Infield, and N.A. McCarty (2017) Bacterial sphingomyelinase is a state-dependent inhibitor of the cystic fibrosis transmembrane conductance regulator (cftr). Sci Rep. 7: 2931.
- Cottrill, K.A., R.J. Peterson, C.F. Lewallen, M. Koval, R.J. Bridges, and N.A. McCarty (2021) Sphingomyelinase decreases transepithelial anion secretion in airway epithelial cells in part by inhibiting cftr-mediated apical conductance. Physiol Reports. 9: e14928.
- Cottrill, K.A., V.D. Giacalone, C. Margaroli, R.J. Bridges, M. Koval, R. Tirouvanziam, and N.A. McCarty (2021) Mechanistic analysis and significance of sphingomyelinase-mediated decreases in transepithelial cftr currents in nhbes. Physiol Reports. 9: e15023.
- Cui, G., K.A. Cottrill, K.M. Strickland, S.A. Mashburn, M. Koval, and N.A. McCarty (2021) Alteration of membrane cholesterol content plays a key role in regulation of cftr channel activity. Front Physiol. 12: 652513.
- Abu-Arish, A., E. Pandzic, J. Goepp, E. Matthes, J.W. Hanrahan, and P.W. Wiseman (2015) Cholesterol modulates cftr confinement in the plasma membrane of primary epithelial cells. Biophysical journal. 109: 85-94.
- Abu-Arish, A., E. Pandzic, D. Kim, H.W. Tseng, P.W. Wiseman, and J.W. Hanrahan (2019) Agonists that stimulate secretion promote the recruitment of cftr into membrane lipid microdomains. The Journal of general physiology. 151: 834-849.
- Bloch, M., I. Raj, T. Pape, and N.M.I. Taylor (2023) Structural and mechanistic basis of substrate transport by the multidrug transporter mrp4. Structure.
- Chen, Y., L. Wang, W.T. Hou, Z. Zha, K. Xu, C.Z. Zhou, Q. Li, and Y. Chen (2023) Structural insights into human abcc4-mediated transport of platelet agonist and antagonist. Nat Cardiovasc Res. 2: 693-701.